4. Host-Guest Complexes with Cyclodextrins |
 |
The molecular shape of cyclodextrins can be roughly described as a hollow truncated cone with no lid or base. This shape is linked to the ability of cyclodextrins to form so-called host-guest complexes with different compounds. The cyclodextrin molecules act as a host to various guest components, which are stabilized in the cyclodextrin cavity as a result of intermolecular interactions. |
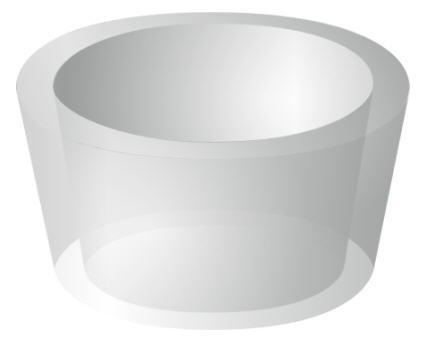 |
Fig. 1.21: Schematic diagram of a cyclodextrin molecule as a hollow truncated cone |
 |
 Van-der-Waals forces form between the cyclodextrin molecules and the guest component. If the guest components contain hydroxyl groups or other groups which are able to form hydrogen bonds, the complex can be significantly stabilized. This is because the intermolecular interactions are strengthened by hydrogen bonding between the guest component and the hydroxyl groups of the cyclodextrin molecule. The stability of a host-guest complex is indicated by the complex formation constant, which is defined as the equilibrium constant as shown in the following reaction: |
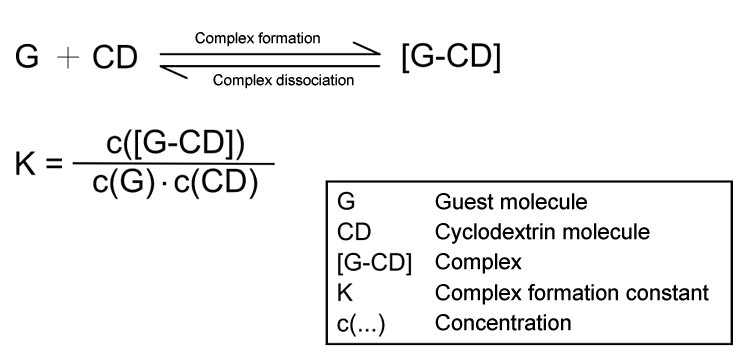 |
Fig. 1.22: The complex formation constant indicates the stability of a host-guest complex |
 |
Guest components that are able to form host-guest complexes with cyclodextrins vary greatly in structure. They range from hydrophobic substances such as alkanes and aromatic hydrocarbons to polar, organic substances and simple anions. This raises the question of what conditions must be present to enable the formation of a cyclodextrin complex. |
 |
 Requirements for the Formation of Host-Guest Complexes |
|
 |
The most important factor is the size of the guest. On one hand, the guest should not be too small, because the intermolecular forces will not form if the distances between the guest and the interior of the cyclodextrin molecule are too great. On the other, if the guest is too large, complex formation cannot take place due to steric hindrance. It must also be considered that it is possible for just parts of a guest molecule to be complexed within the cavity. The size of the cavity in relation to the size of the guest is also critical for complex formation. The table shows how the size of the guest molecule affects complex formation, using aromatic hydrocarbons as an example. |
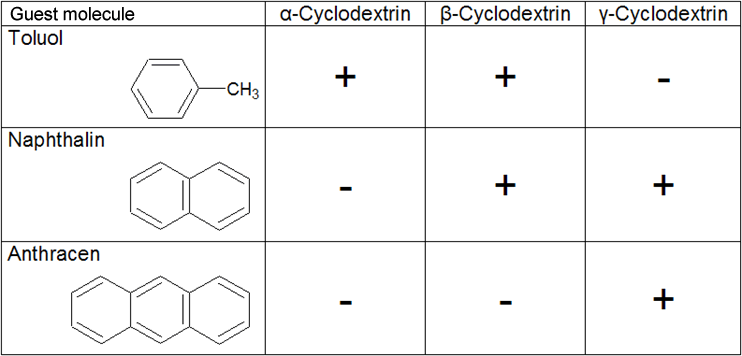 |
Table 1.5: Ability to form complexes with aromatic hydrocarbons |
 |
This selectivity in terms of complex formation is exploited in industrial production by using selective precipitating agents for each cyclodextrin.
The charge and polarity of the guest component also influence complex formation. In general, it can be said that highly water-soluble, highly hydrophilic and therefore highly hydratable guests are not suitable for complex formation. The degree to which the charge on the guest favors complex formation cannot be accurately predicted. For example, in the complexation of p-nitrophenolate, the charge produces a more stable complex compared with that of p-nitrophenol. The reason is the greater dipole moment of the anion. The guest p-nitrophenolate is therefore able to induce a stronger dipole in the cyclodextrin molecule. The dipole-dipole interactions are greater, and the complex is stabilized as a result.
The factors affecting complex formation must be re-evaluated and experimentally studied for each guest component. |
 |
 The Forces Driving Complex Formation |
 |
So far, we have mentioned the factors necessary or advantageous for complex formation. We will now consider the forces driving the formation of host-guest complexes. According to the second law of thermodynamics, a reaction is spontaneous when the Gibb’s free energy ΔG < O. According to the equation ΔG = ΔH – T*ΔS, the driving force derives from the system’s trying to minimize enthalpy and maximize entropy.
Although the occurrence of intermolecular interactions between host and guest lowers the enthalpy of the system, the relatively weak interactions alone cannot represent the force driving complex formation. Water appears to play a crucial role in complex formation. For example, benzoic acid does not form complexes in organic solvents such as benzene or chloroform. However, when water is the solvent, the benzoic acid readily forms complexes with cyclodextrin. The presence of water has both an enthalpic and an entropic affect on the formation of host-guest complexes. During complex formation, the water molecules present in the cavity are exchanged for a guest component. The size of the cyclodextrin molecule determines the number of water molecules present in the cavity (see Table 1.6 ). The entropy in the system increases when the water molecules are displaced by a guest molecule, and this favors complex formation. |
 |
|
α-Cyclodextrin |
β-Cyclodextrin |
γ-Cyclodextrin |
Hydrate formation |
α-CD * 6 H2O |
β-CD * 11 H2O |
γ-CD * 15.7 H2O |
Number of water molecules in the cavity |
1 – 2 |
6 |
8.8 |
|
 |
Table 1.6: Number of water molecules in the cavity of natural cyclodextrins |
 |
In addition to the entropic effect, there is also a gain in enthalpy. Even when the cyclodextrin molecules are in the crystalline state, they contain water molecules. These water molecules are limited in their ability to form hydrogen bonds due to restrictions imposed by the non-polar inner side of the cyclodextrin molecule and the small number of water molecules in the immediate environment. The water molecules in the cavity therefore have a higher enthalpy than the water molecules in the solvent. Expulsion of these water molecules from the cavity of the cyclodextrin molecule and the complete formation of hydrogen bonds lowers the enthalpy and thus further benefits complex formation. |
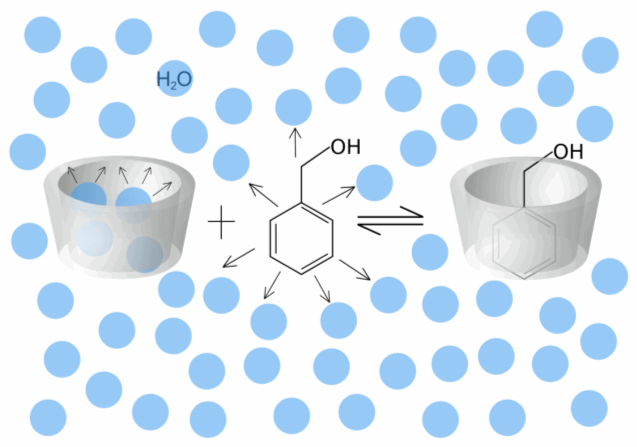 |
Fig. 1.23: Schematic diagram of the complex formation of benzyl alcohol, in which the arrows indicate repulsive forces |
 |
An additional enthalpic effect must be considered during complex formation, since complexation may lead to an energy gain due to relaxation of the ring strain in the cyclodextrin molecule. This effect can be observed in particular with α-cyclodextrin, since the twisting of a glucose unit in the α-cyclodextrin molecule is canceled during complex formation.
Solvation processes also influence complex formation. Complex formation is accompanied by a gain in energy if the guest molecule cannot be hydrated in water (see Fig. 1.23), because the repulsive interactions between the guest molecules and the water molecules cease when the guest molecule forms a complex with cyclodextrin.
In conclusion, it can be said that several factors affect the formation of a host-guest complex. However, it is not possible to establish a hierarchy of influencing factors as the effects also exert influences on each other and vary from one guest molecule to another. |
 |
 Detecting the Formation of Cyclodextrin Complexes |
 |
Various methods can be used to study the complexation of a guest molecule in cyclodextrins. 1H-NMR spectroscopy can provide direct evidence of complex formation, since the hydrogen atoms at carbon atoms 3 and 5 of each glucose unit in the cyclodextrin molecule are shifted relative to their positions in the spectra of the pure cyclodextrins. |
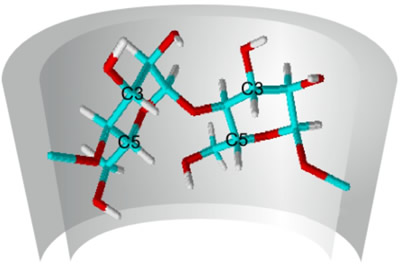 |
Fig. 1.24: The intermolecular interactions in the host-guest complex shift the signals of the inwardly facing hydrogen atoms in the cyclodextrin molecule |
 |
UV-VIS spectroscopy is an alternative method of studying host-guest complexes with cyclodextrins that can be used when complexation modifies the absorption spectrum of the guest molecule. Modified reactivity of the guest component can also be used to detect complex formation, since the guest molecule is shielded from reaction partners by the formation of a host-guest complex.
The stoichiometry of the host-guest complex can also be determined by analytical methods. So far we have not considered the fact that a guest molecule can be complexed by several cyclodextrin molecules.
For example, the retinol complex has a 2:1 ratio of cyclodextrin to retinol (see Fig. 1.25). |
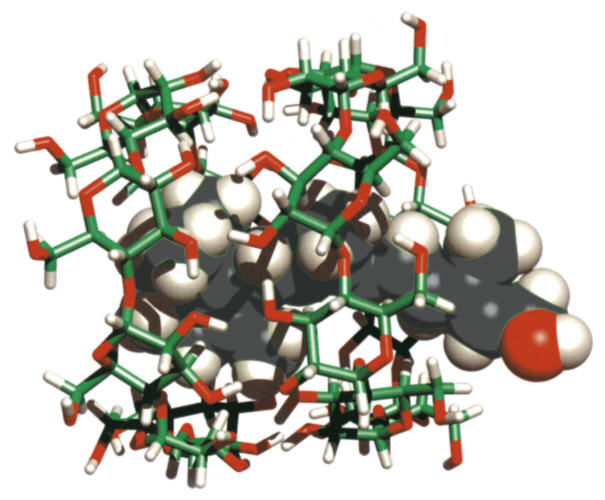 |
Fig. 1.25: Two cyclodextrin molecules (rods) complexing one retinol molecule (solid balls) |
 |
 Effect of Complex Formation on the Guest Components |
 |
The complexation of guest molecules has been relatively well researched due to the very diverse range of applications. The formation of cyclodextrin complexes can modify the physical and chemical properties of the guest molecules.
In the case of readily volatile substances, such as aromas, rapid evaporation can be prevented by complexation with cyclodextrins, because the intermolecular interactions considerably lower the vapor pressure of the guest molecules. Complexation can additionally protect oxidation-sensitive guest molecules, such as the omega-3,6 fatty acids, from degradation by atmospheric oxygen. The length of the fatty acid groups requires several cyclodextrin molecules to be involved in the complexation of a single molecule (see Fig. 1.26). |
 |
Fig. 1.26: The long alkyl chains require several cyclodextrin molecules for complexation |
 |
The breakdown of guest molecules by photo-oxidative degradation can also be delayed significantly, and this protects the guest molecules from light. The popularity of cyclodextrins as subjects of pharmaceutical research is largely due to the fact that complexation in cyclodextrins can increase the water solubility and therefore also the bioavailability of pharmaceutical substances.
|
 |
 Production of Host-Guest Complexes |
 |
Various methods can be used to produce host-guest complexes, provided that they take into account the equilibriums shown in Figure 1.27. |
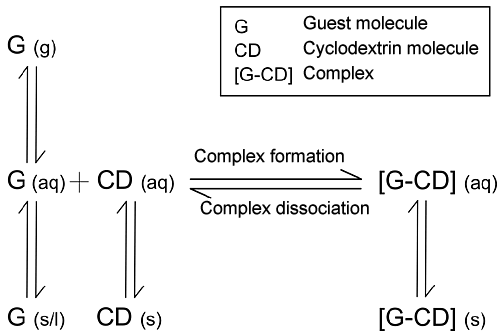 |
Fig. 1.27: Equilibriums involved in complex formation |
 |
When a complex is formed from a solution, the guest component is added to a virtually saturated solution in an equimolar ratio. With guest components that tend to be more hydrophobic, the complex forms as a precipitate during mixing. The solid is filtered, washed and then dried under vacuum. Readily volatile guest compounds should not be dried under vacuum, since the equilibrium responds to the removal of the guest by constantly dissociating the complex. If the complex is water-soluble, a non-polar solvent can be added to facilitate precipitation of the complex. Complex formation from a suspension is a suitable method for β-cyclodextrin in particular, since this is sparingly water-soluble. Here, too, the guest component is added in an equimolar ratio and agitated for 12 to 24 hours. Preparation then continues as described above. |
 |
References:
- Tausch, M.; von Wachtendonk, M.; Chemie SII Stoff-Formel-Umwelt, C.C.Buchner Bamberg, 1993, S. 80-82
- Szejtli, J.; Osa, T.; Comprehensive Supramolecular Chemistry. Volume 3 Cyclodextrins, Elsevier Science Ltd. Oxford, 1996, S. 21-22
- Bender, M.L.; Komiyama, M.; Reactivity and Structure Concepts in Organic Chemistry. Volume 6 Cyclodextrin Chemistry, Springer-Verlag Berlin, 1978, S. 10-23
- Wacker Chemie AG; Komplexbildung mit natürlichen Cyclodextrinen CAVAMAX® (www.wacker.com/internet/webcache/de_DE/PTM/BioTec/Cyclodextrins)
- Szejtli, J.; Chem. Rev., 1998, 98, 1743-1753
- Gröger, M.; Kretzer, E.K.; Woyke, A.; Reader mit Hintergrundinformationen zum Thema Cyclodextrine, Siegen, 2001, S. 17
- Szejtli, J.; Pure Appl. Chem., 10, 2004, 76, 1825-1845
- Szejtli; J.; Cyclodextrins and their inclusion complexes, Akadémiai Kiadó Budapest, 1982, S. 97
|
 |
| Home | Wuppertal University | WACKER | Didactic Dept. | Supp. Info | Experiments | Media | Contact | |