1. Experiment is contained in the WACKER's Experimental Kit. |
No |
2. Experimental procedure has been modified |
/ |
3. A separate experimental procedure has been devised |
Yes |
4. Video clip available |
No |
5. Flash animation available |
No |
 |
UV Resistance of Silicone Rubber Compared
With That of Other Elastomers |
 |
 1
Materials, Chemicals, Time Needed |
 |
 |
 |
- High-pressure mercury lamp (e.g. Hanau TQ 150 with quartz
- cooling
shaft; see table opposite for spectrum)
- Cardboard
- Spring balance + press
- Scotch tape
- Laboratory platform
- Glass rod
- Petri dish
- Microscope
- Carpet knife
- Stop-watch
- Various rubber specimens, e.g. bicycle inner tube, rubber
tubing (laboratory tubing), natural rubber
Various silicone rubber specimens from WACKER's Experimental Kit, e.g. HTV(b), HTV(s) and HTV(w).
Preparation and examination of each specimen will take around
15 minutes. Irradiation alone will take a further 30 minutes.
Overall, ¾ of an hour should be allowed for each specimen. |
|
TQ 150
naked emitter |
l(nm) |
Radiation Flux |
Mole quanta per hour |
238/40 |
1.0 |
8 |
248 |
0.7 |
5 |
254 |
4.0 |
30 |
265 |
1.4 |
11 |
270 |
0.6 |
5 |
275 |
0.3 |
2 |
280 |
0.7 |
6 |
289 |
0.5 |
4 |
297 |
1.0 |
9 |
302 |
1.8 |
17 |
313 |
4.3 |
41 |
334 |
0.5 |
5 |
365 |
6.4 |
71 |
390 |
0.1 |
1 |
405/8 |
3.2 |
39 |
436 |
4.2 |
55 |
492 |
0.1 |
1 |
546 |
5.1 |
84 |
577/49 |
4.7 |
82 |
Source: Schule Chemie System SCS UV-Tauch-
lampenreaktor für Schulversuche, M. Tausch |
|
 |
 2
Procedure and Observations |
 |
Naked UV light must be avoided at
all costs because it can quickly damage eyes and skin. The irradiation
step may lead to the formation of ozone. A fume cupboard and
UV protection (cover with cardboard or aluminum foil) must be
used when working with the quartz lamp! |
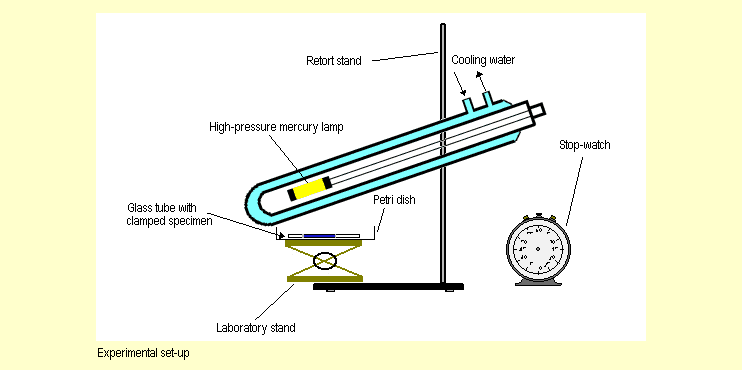 |
 Set
up the apparatus in the fume cupboard as shown in the diagram
above. Using the laboratory stand, place the petri dish as close
as possible to the radiation source. To ensure that comparable
results are obtained, do not alter the distance between the petri
dish and the radiation source during the series of experiments. |
 |
 |
Keep the apparatus covered with an appropriately
shaped piece of cardboard during irradiation.
Before irradiation, cut each elastomer specimen
into a rectangle and test its tensile strength both manually
and under a tensile force of 10N.
Then tape the specimen to the glass rod and stretch it under
a tensile force of 10N. Place the specimen face up on the
petri dish (right photograph + sketch). Irradiate the specimen
for 30 minutes and then examine it for mechanical, macroscopic
and microscopic changes.
Repeat this procedure for all the specimens. |
|
 |
The following reproducible observations were made
in reference experiments: |
 |
 1)
Natural rubber: (force = 1 N, width = approx. 1 cm)
The natural rubber specimen was only stretched under a tensile
force of 1 N, as otherwise it would have become too long.
The properties of the natural rubber were severely affected by
the radiation.
Macroscopically, the specimen tears under load and turns whitish.
The surface of the specimen is tacky.
Examination under the microscope shows that irradiation has led
to the formation of a net-like structure consisting of black
lines (see photo 2).
Prior to irradiation, the specimen had been transparent, except
for a few dirt particles (see photo 1). |
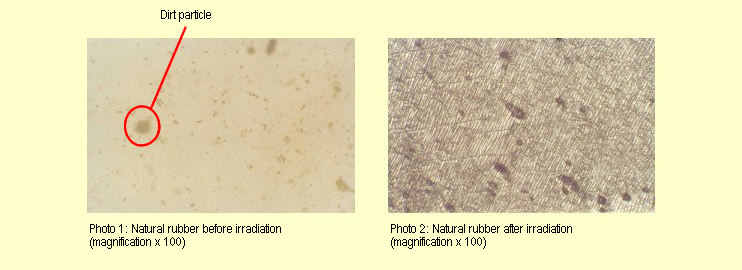 |
 2)
Bicycle inner tube: (force = 10 N, width = approx. 0.9 cm)
The 30 minutes of irradiation failed to produce any macroscopic,
microscopic (see photos 3 and 4) or mechanical changes in the
bicycle inner tube. |
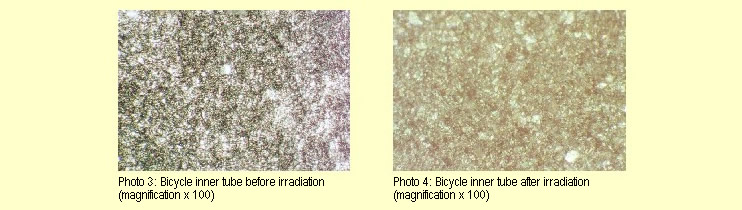 |
The differences in the brightness of the two photos
stem from differences in light intensity when the photos were
taken.
3) Silicone rubber HTV(b): (force = 10 N, width = approx.
0.5 cm)
The 30 minutes of irradiation failed to produce any macroscopic,
microscopic (see photos 3 and 4) or mechanical changes in
the HTV(b) specimens.
Unfortunately, no photomicrographs could be taken as the
nature of the specimens made it impossible to focus the lens.
 4)
Silicone rubber HTV(s): (force = 10 N, width = approx.
0.5 cm)
The 30 minutes of irradiation failed to produce any macroscopic, microscopic
(see photos 5 and 6) or mechanical changes in the HTV(s) specimens.
|
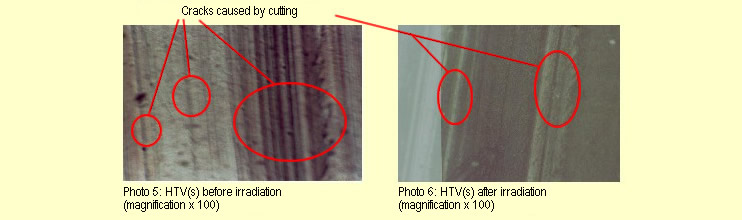 |
The different appearances of the two photomicrographs
stem from differences in light intensity and the different structure
(parallel stripes) caused by cutting to size. The stripes visible
under the microscope were already present before irradiation
and were caused by cutting the specimen.
 5)
Silicone rubber HTV(w): (force = 10 N, width = approx. 0.5
cm)
The 30 minutes of irradiation failed to produce any macroscopic,
microscopic (see photos 7 and 8) or mechanical changes in the
HTV(w) specimens.
|
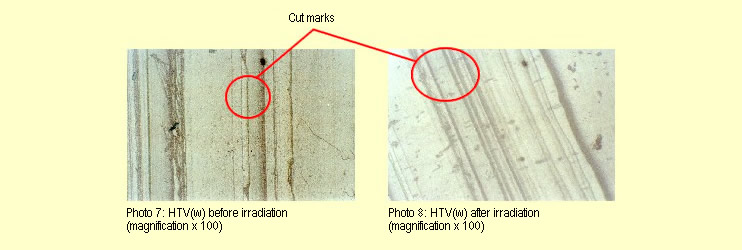 |
The different appearances of the two photomicrographs
stem from differences in the light intensity and the different
structure (parallel stripes) caused by cutting to size. The
stripes visible under the microscope were already present before
irradiation and were caused by cutting the specimen.
 6)
Rubber tubing (laboratory tubing): (force = 10 N, width =
approx. 1 cm)
Irradiation of the rubber tubing specimens produced different
results. In most of the experiments, the microscopic structure
of the specimens was altered by the irradiation to the extent
that, following irradiation, longitudinal lines and signs of
a lattice structure are visible (see photos 9 and 10).
In several cases, however, there were no changes.
None of the rubber tubing specimens showed either macroscopic
or mechanical changes.
|
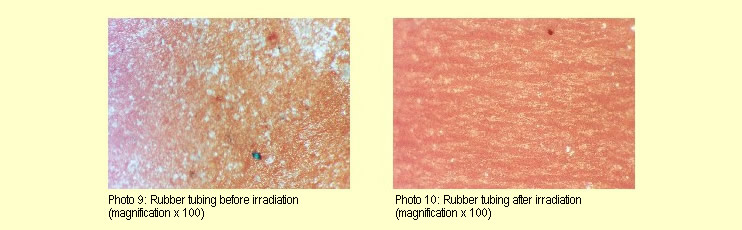 |
A possible reason for the different
observations may be the different tensile stresses placed on
the various specimens. Since the specimens are not exactly the
same size and since the Scotch tape yields slightly, the tensile
force varies somewhat between the different specimens and only
approximates to 10 N.
Closer examination revealed that the microscopically observed
changes depended heavily on the distance between the specimen
and the radiation source.
 The
experimental results are summarized in the following table:
|
 |
Specimen |
Observation
after 30 minutes’ irradiation |
Natural rubber |
Natural rubber undergoes
extensive changes in macroscopic, microscopic and mechanical
properties when exposed to UV radiation. |
Bicycle inner tube |
No identifiable changes |
Rubber tubing (laboratory
tubing) |
Microscopic changes
were found in the rubber tubing. However, there were no
changes in the macroscopic and mechanical properties. |
HTV(b) |
No identifiable changes |
HTV(s) |
No identifiable changes |
HTV(w) |
No identifiable changes |
|
 |
 3
Discussion of Results
|
 |
The experimental results are
in good agreement with literature values concerning the response
of different macromolecular compounds to irradiation with UV
light.
Whereas UV radiation does not affect silicones, it does affect
rubber, which contains cis-1,4-polyisoprene. Its elastic properties,
in particular, are damaged. These changes are chemical by nature
and are induced by the high-energy UV radiation (see also 5
Supplementary Information). Even visible light, and UV
light much more so, causes photochemical oxidation (photooxidation)
of natural rubber in the presence of oxygen. This starts off
with a reduction in molar mass (i.e., fragmentation of the
macromolecules) followed by crosslinking of the fragments.
The material turns soft and its surface becomes tacky. A brittle
layer forms later. The light causes homolytic scission of several
C-H bonds in the macromolecules that make up the natural rubber
(scission occurs mainly at allyl C-H bonds, i.e. those in α-position to the carbon=carbon double
bonds). This homolysis generates free-radicals that initiate
chain reactions in which oxygen is also involved. This is called autoxidation. The primary products of autoxidation are fragments
of the original macromolecules, which have now been converted
into hydroperoxides (R-O-OH) or peroxides (R-O-O-R) (see reaction
mechanisms). These primary products are generally unstable and
disintegrate into other products. |
 |
 In
addition to autoxidation, natural rubber can also undergo photochemical
[2+2] cycloaddition because the polymer chains often contain
carbon=carbon double bonds and because these chains are close
together.
This reaction proceeds as shown below for the example of dimerization
of two cis-1,4-polyisoprene molecules (natural rubber): |
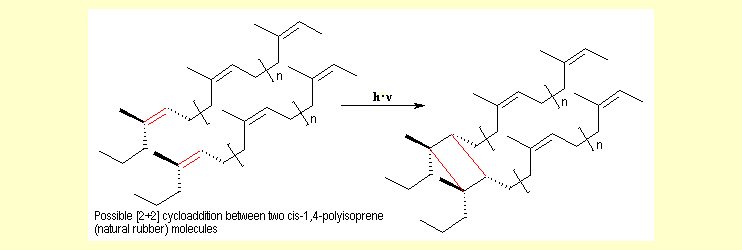 |
 Autoxidation
and [2+2] cycloaddition are behind the changes observed both
in the natural rubber and in the rubber tubing (laboratory tubing),
which, as the manufacturer says, consists of a mixture of natural
rubber and butadiene-styrene rubber (BSR). The changes are not
as pronounced in this specimen as they are in the natural rubber
because the rubber tubing specimen is thicker and has been cured
more fully. If this specimen were to be exposed to the radiation
for longer, there would be greater changes in the properties.
However, since the experiment is designed to be performed during
one lesson, the effects of longer exposure to the radiation were
not examined.
There are two reasons why the bicycle inner tube is resistant
to UV radiation: First, the inner tube is made from butyl rubber,
which is a copolymer consisting of just 1 to 3 % isoprene (2-methyl
butadiene) and 97 to 99 % isobutene. Butyl rubber therefore contains
far fewer C=C double bonds than does natural rubber. This means
that there are also far fewer groups of atoms that can undergo
autoxidation and [2+2]-cycloaddition. Second, the inner tube
contains added carbon black, which improves its mechanical properties
and wear resistance. Carbon black absorbs light of any wavelength
(hence its black color) and thus reduces the number of photons
that cause degradation. Even rubber that contains a higher proportion
of natural rubber (cis-1,4-polyisoprene) can be made more resistant
to UV radiation by adding carbon black. |
 |
 4
Tips and Comments
|
 |
- This experiment shows the pupils that irradiation
can cause photochemical reactions in elastomers that will
adversely affect their properties. The conditions employed
in the experiment
are much more drastic (short-wave, intense UV radiation)
than the rubber would experience in reality. It thus is typical
of
the experiments used to test the properties of materials.
- There
are simpler experiments than this one for introducing
pupils to the interaction between light and materials, as well
as
photochemical reactions. However, the results presented
here could be used
in project work and/or young scientist experiments and
be supplemented with further experiments.
|
 |
 5
Supplementary Information
|
 |
The starting point for the
chemical reactions of polymers on exposure to visible and UV
light is
the electronic transition induced by absorption of the electromagnetic
radiation (see also http://www.chemiedidaktik.uni-wuppertal.de/ > Licht
und Farbe, Licht und Energie).
Under normal conditions, i.e. at room temperature and away from
light, molecules are in their base electron
configuration (see
Fig. 1). The permitted energy levels in the molecule are each
fully occupied from the bottom up to the top with pairs of electrons.
In molecular orbital theory, the highest occupied energy level
is termed the HOMO (highest occupied molecular
orbital). Figure
1 illustrates occupancy of the HOMO in the form of two opposing
arrows to signify that the electrons have opposite spin. The
next energy level above the HOMO permitted for the molecule but
not occupied with electrons when the molecule is in the ground
state is called the LUMO (lowest unoccupied
molecular orbital).
By absorbing a photon of energy , i.e. precisely the energy difference
between the LUMO and the HOMO, the molecule shifts from the ground
state to its excited state. When the electron jumps from the
HOMO to the LUMO, a process that takes approx. 10-15 s, it retains
its spin.
 The
excited state is said to be a singlet state (see Fig. 2; the
term singlet will not be explained here). Having reached the
excited singlet state, the system (the excited molecule) can
return to its ground state within approx. 10-9 s again by emitting
a light quantum (observed as fluorescence) or emitting heat.
A third possibility is for it to enter into a chemical reaction,
e.g. homolytic scission of a bond. Homolysis of a C-H bond,
the first step in autoxidation, is an example of this type
of photochemically induced reaction in the excited singlet
state. |
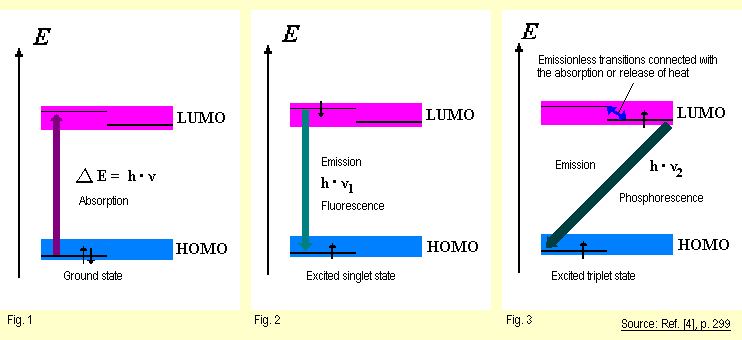 |
 Under
certain conditions, which will not be explained here, the excited
electron can reverse its spin. The resultant excited triplet
state (see Fig. 3) has a much longer life time of 10-3 to
101 s than the singlet state. The reason is that a
return from the excited triplet state to the ground state is
forbidden by quantum mechanics and thus is much more unlikely
than a return from the excited singlet state to the ground state.
When excited molecules in the triplet state emit light quanta
and so become deactivated, the observer sees this as phosphorescence,
which lasts longer than fluorescence. Because of its relatively
long life time, an excited molecule in the triplet state can
also participate in bimolecular reactions, i.e., in reactions
that require a collision between it and another molecule. In
the discussion above of the photochemical reactions occurring
in natural-rubber elastomers (cis-1,4-polyisoprene), triplet
states are likely also involved, especially in the bimolecular
reaction steps. |
 |
 6
References |
 |
- M. Tausch, M. von Wachtendonk (editors), CHEMIE S II, STOFF-FORMEL-UMWELT,
C.C. Buchner, Bamberg (1993), (1998), S. 297 - 302
- D. Wöhrle, M. W. Tausch, W.-D. Stohrer, PHOTOCHEMIE
- Konzepte, Methoden, Experimente, Wiley-VCH, Weinheim (1998)
|
 |
|
|